eEF2K
Eukaryotic Elongation Factor 2 Kinase
Introduction
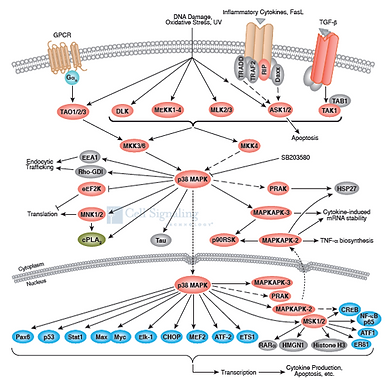
Eukaryotic elongation factor-2 kinase (eEF2K) is a serine/threonine kinase that participates in calcium/calmodulin-dependent signal transduction pathways (1). Its primary function is to phosphorylate its (only known) substrate: the protein eukaryotic elongation factor-2 which inhibits the elongation phase of translation and halts protein synthesis (2). A mode by which gene expression can be regulated is through controlling mRNA translation (i.e. protein synthesis). Protein synthesis proceeds in three primary steps: initiation, elongation and termination and requires a high energy input (4 ATP molecules consumed per amino acid) with the elongation phase utilising most of the energy provided. Accumulating evidence suggests that elongation control is particularly important in the cell’s response to stressful conditions.
eEF2K is a member of the ‘α-kinase’ atypical protein kinase family but barely possesses sequence homology or structural similarity with the main protein kinase family. It is encoded by the EEF2K gene, which is located on chromosome 16 (1) and shows to be conserved throughout evolution with 179 species having orthologues (3). The eEF2K structure consists of various domains: at the N-terminus, there is the calcium/calmodulin binding domain followed by the α-kinase catalytic domain. Adjacent to the catalytic region lies the linker region, embedded with phosphorylation sites that regulate enzymatic activity. This is followed by four α-helical SEL1-like motifs that allow protein-protein interactions (4). At the C-terminus lies a critical, highly conserved region for eEF2 binding and phosphorylation.

Activation of eEF2K isn’t well elucidated but it is thought to involve the binding of calcium/calmodulin to its cognate binding site N-terminal to the catalytic domain. This causes removal of auto-inhibitory components from the activate site and allows eEF2K to undergo auto-phosphorylation. As mentioned, particular residues modulate enzymatic activity such as the Thr348 residue, when phosphorylated, allows the protein to attain its optimum conformation thus enhancing its kinase activity (5, 6).
eEF2K activity is regulated by other mechanisms including phosphorylation by several upstream enzymes within signalling pathways. Mammalian target of rapamycin complex 1 (mTORC1), a sensor of the cell’s bioenergetics status, is known to stimulate protein synthesis and ribosomal production therefore mTORC1 mediated phosphorylation represses eEF2K. On the other hand, other proteins such as cAMP-dependent protein kinase A (PKA) and AMP-activated protein kinase (AMPK) upregulate eEF2K activity to shunt the energy consumed by protein synthesis when cells are short of nutrients and ATP.
Diagram constructed by group on draw.io
Therefore it appears that eEF2K confers cytoprotection in times of stress such as oxygen and nutrient deprivation. Studies have shown that eEF2K upregulation can underlie tumour growth thus making the enzyme an attractive therapeutic target in cancer. Accumulating evidence suggests that eEF2K is involved in an array of other activities such as autophagy induction (8) and muscle contraction (9). In the brain, eEF2K appears to play a principal role as its expression is associated with synaptic plasticity and in consolidating mechanisms involved in learning and memory (10-12). The remarkably diverse nature of eEF2K emphasises its significance in cellular processes and if better comprehended, it can give new perspectives on both health and pathogenesis.
References
1. Zhu H, Yang X, Liu J, Zhou L, Zhang C, Xu L et al. Eukaryotic elongation factor 2 kinase confers tolerance to stress conditions in cancer cells. Cell Stress and Chaperones. 2014;20(2):217-220.
http://www.ncbi.nlm.nih.gov/pubmed/25248493
2. Kenney J, Moore C, Wang X, Proud C. Eukaryotic elongation factor 2 kinase, an unusual enzyme with multiple roles. Advances in Biological Regulation. 2014;55:15-27.
http://www.ncbi.nlm.nih.gov/pubmed/?term=24853390
3. National Center for Biotechnology Information. ‘EEF2K eukaryotic elongation factor 2 kinase [Homo sapiens (human)]’. Available from: http://www.ncbi.nlm.nih.gov/gene?Db=gene&Cmd=DetailsSearch&Term=29904. [Accessed: 8th March 2016].
4. Mittl P, Schneider-Brachert W. Sel1-like repeat proteins in signal transduction. Cellular Signalling. 2007;19(1):20-31.
http://www.ncbi.nlm.nih.gov/pubmed/16870393
5. Pyr Dit Ruys S, Wang X, Smith E, Herinckx G, Hussain N, Rider M et al. Identification of autophosphorylation sites in eukaryotic elongation factor-2 kinase. Biochemical Journal. 2012;442(3):681-692.
http://www.ncbi.nlm.nih.gov/pubmed/22216903
6. Tavares C, Ferguson S, Giles D, Wang Q, Wellmann R, O'Brien J et al. The Molecular Mechanism of Eukaryotic Elongation Factor 2 Kinase Activation. Journal of Biological Chemistry. 2014;289(34):23901-23916.
http://www.ncbi.nlm.nih.gov/pubmed/25012662
7. Wang X, Regufe da Mota S, Liu R, Moore C, Xie J, Lanucara F et al. Eukaryotic Elongation Factor 2 Kinase Activity Is Controlled by Multiple Inputs from Oncogenic Signaling. Molecular and Cellular Biology. 2014;34(22):4088-4103.
http://www.ncbi.nlm.nih.gov/pubmed/25182533
8. Wu H, Zhu H, Liu D, Niu T, Ren X, Patel R et al. Silencing of Elongation Factor-2 Kinase Potentiates the Effect of 2-Deoxy-D-Glucose against Human Glioma Cells through Blunting of Autophagy. Cancer Research. 2009;69(6):2453-2460.
http://www.ncbi.nlm.nih.gov/pubmed/19244119
9. Rose A, Alsted T, Jensen T, Kobberø J, Maarbjerg S, Jensen J et al. A Ca2+-calmodulin-eEF2K-eEF2 signalling cascade, but not AMPK, contributes to the suppression of skeletal muscle protein synthesis during contractions. The Journal of Physiology. 2009;587(7):1547-1563.
http://www.ncbi.nlm.nih.gov/pubmed/19188248
10. Sakagami H, Nishimura H, Saito R, Kondo H. Transient up-regulation of elongation factor-2 kinase (Ca2+/calmodulin-dependent protein kinase III) messenger RNA in developing mouse brain. Neuroscience Letters. 2002;330(1):41-44.
http://www.ncbi.nlm.nih.gov/pubmed/12213630
11. Liu-Yesucevitz L, Bassell G, Gitler A, Hart A, Klann E, Richter J et al. Local RNA Translation at the Synapse and in Disease. Journal of Neuroscience. 2011;31(45):16086-16093.
http://www.ncbi.nlm.nih.gov/pubmed/22072660
12. Takei N, Kawamura M, Ishizuka Y, Kakiya N, Inamura N, Namba H et al. Brain-derived Neurotrophic Factor Enhances the Basal Rate of Protein Synthesis by Increasing Active Eukaryotic Elongation Factor 2 Levels and Promoting Translation Elongation in Cortical Neurons. Journal of Biological Chemistry. 2009;284(39):26340-26348.